For the Public - Research Highlights |
Physics Highlights from the DØ Experiment (1992--1999)
Fermi National Accelerator Laboratory, Batavia, Illinois, U.S.A.
5. QCD PHYSICS
Quantum Chromodynamics (QCD) is the part of the Standard Model that describes the strong interaction responsible for the nuclear force. The
quarks that make up the proton and all hadrons interact with gluon force carriers by virtue of their "color" quantum number. Though the proton can be viewed simplistically as a collection of three quarks, when examined closely, it reveals substantially more complex internal structure. The additional quarks and gluons appear with increasing magnification, or at larger momentum transfers, commensurate with smaller distances, and
are described by phenomenological functions called parton distribution functions (PDFs). These PDFs are derived from data, and therefore have
uncertainties that have to be taken into account in any QCD-based prediction. Moreover, the basic coupling strength between quarks and gluons, as, decreases as the momentum transfer in a process increases. Hence, perturbative calculations of strong-interaction processes become more precise at large values of the square of four-momentum transfer (q2), whereas at low q2 such calculations are extremely difficult, and often not
reliable.
Because of the excellent coverage for jets provided by the calorimeter, DØ has made detailed and accurate measurements of strong interaction
processes that test the predictions of QCD in many domains. We have already remarked on the great success of QCD in predicting the
production of top quarks, and we focus here on only a few processes that pertain to the production of jets (quarks and gluons) and W and Z
bosons.
The elastic scattering of quarks (or gluons) within the colliding proton and antiproton resembles classic Rutherford scattering of alpha particles by gold nuclei. Both processes are well described by the exchange of a spin 1 quantum (a photon or a gluon) for the case when the interacting
objects display no substructure. The inclusive production of jets at very large transverse energy (ET) can be calculated with confidence in
QCD, given knowledge of the PDFs. Using Run 1 data, DØ has published the inclusive jet ET spectrum in the range 60 < ET < 560 GeV. In this
measurement, jets were detected in the central region of the detector. Figure 8 shows the observed cross section, which drops by six orders of
magnitude over the measured range. Taking account of the statistical and systematic uncertainties, DØ finds that the QCD prediction, including
its higher order corrections (and using standard PDFs), agrees well with the data. This result attracted considerable attention because CDF had
published an inclusive jet cross section, which showed possible excess above theoretical predictions at the high-ET end of the spectrum. If such
excess were confirmed, it could be interpreted as providing evidence for quark compositeness or the presence of other new physics beyond the
Standard Model. The DØ result showed that Standard Model calculations do not need to be augmented with new physics beyond expectations
from QCD.
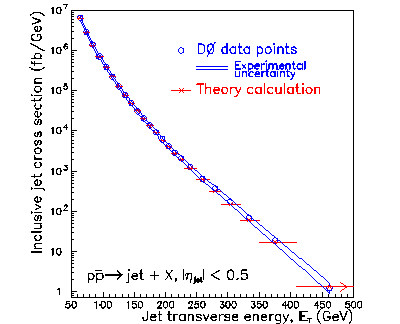
Fig. 8: The measured DØ inclusive jet cross section compared with QCD calculations.
The inclusive jet cross section was also measured during a special Tevatron run at lower center of mass energy of 630 GeV (where the earlier
CERN measurements had been made). Taking the ratio of the inclusive jet cross sections at 630 and 1800 GeV cancels many experimental and
theoretical uncertainties. DØ measured this ratio to be about 20% lower than expected. However, better agreement can be obtained if the
energy scales for the perturbative calculations are defined differently at the two center of mass energies.
Using the two highest-ET jets among those reconstructed in any event; DØ calculated an invariant mass to search for possible new particles that
might decay into two jets. Such a state would appear as a bump above the smooth background of ordinary QCD production. The slope of the
falling dijet mass distribution is also sensitive to possible substructure of quarks and gluons. DØ has published the dijet mass spectrum for the
range of 200 to 1,400 GeV, and found no structures. A quantitative limit on quark compositeness was determined from the shape of this
distribution. A possible substructure can be characterized by a mass-scale parameter L, corresponding to bound states of any subunits within
quarks. For L < 2.4 TeV, the slope of the predicted mass spectrum would be inconsistent with DØ's measured result. This limit on L indicates
that there is no substructure within quarks or gluons down to the attometer scale (10-18 m), and is the most stringent limit on quark substructure
determined by experiments to date.
W and Z bosons are created primarily through the annihilation of valence quarks and antiquarks, and so a comparison of measured W and Z
production cross sections with theoretical predictions provides test of QCD that is complementary to jet production. Using W and Z decays in
both electron and muon channels; DØ has measured the ratio of the W/Z cross section multiplied by their respective branching fractions to
leptons. The resulting ratio of 10.49 ± 0.25 is in excellent agreement with the QCD calculation to order as2 of R = 10.73 ± 0.11, where the
theoretical uncertainty stems from choice of input PDF and variations due to the uncertainty in MW and energy reference-scale factors used in
the theory. The measurement of R was also used to extract the total decay width of the W (GW = 2.152 ± 0.066 GeV), and to determine that no
more than 8% of W decays could proceed into unexpected final states. DØ has also measured the transverse momentum spectra for the
production of the W and Z bosons. The comparison of these distributions is the most sensitive to non-perturbative effects from multiple gluon
radiation present in low-q2 QCD.
In data that contain at least two high-ET jets, DØ has observed that a small fraction of events have the striking feature of sizeable gaps in energy deposition between the two jets, or between jets and the beam direction. The gaps are characterized by the absence of particles in extended regions of polar angle in the tracking detectors, calorimeters or forward trigger counters. Such events are termed "rapidity-gap" events (the
rapidity variable is related to the polar angle), and fall into three topological categories: jet-gap-jet, gap-jet-jet, and gap-jets-gap, depending on the location of the gaps in the detector. Events in the first two categories (jet-gap-jet and gap-jet-jet) were observed about 1% of the time of events with similar jet topologies. Events of the third category (gap-jets-gap) were observed about 1% of the time of events in category 2 (gap-jet-jet). Dijet events of all three topologies have been observed at both 1800 GeV and 630 GeV. Similar topologies have also been reported at the e-p collider experiments at HERA in Hamburg Germany.
Explanations for the gap events are based on the supposition of the existence of a color-free object called the Pomeron. The Pomeron has long
been postulated as the exchanged object and force carrier responsible for elastic and diffractive scattering of two hadrons. The colorless
property of the Pomeron is used to explain the presence of rapidity gaps. Ordinary hadrons are produced due the color carried by their
constituents, hence their emission from the color-free Pomeron is suppressed. The jets produced in these events have ET distributions similar to those in standard QCD (quark and gluon exchange) processes. This leads to the view that the Pomeron may have an internal structure, consisting
at least partly of normal quarks and gluons arranged in such a way as to make the Pomeron colorless. DØ's study of rapidity-gap events will be
enhanced during Run 2, when a set of detectors very close to the beams will enable the experiment to intercept diffractively scattered beam
particles on either side of the interaction point. These detectors will provide the full kinematic reconstruction of certain gap-jet-jet and
gap-jets-gap topologies, shedding more light on the Pomeron's structure and dynamics.
< Back Next > - PHYSICS OF THE BOTTOM QUARK
Return to For the Public main page
|