For the Public - Research Highlights |
Physics Highlights from the DØ Experiment (1992--1999)
Fermi National Accelerator Laboratory, Batavia, Illinois, U.S.A.
4. ELECTROWEAK PHYSICS
One consequence of the unification of the electromagnetic and weak forces was the prediction of the existence of two new particles: the W and
Z gauge bosons. After several years of search by experiments around the world, two collaborations at CERN, using the world's most powerful
accelerator at the time, announced in 1983 the first direct observation of these elusive particles. With a total of ten W bosons and four Z bosons,
the experiments measured the masses of the particles to be ~ 80 GeV and ~ 90 GeV respectively, with an uncertainty of 5–10 %. While the
number of events was relatively small, the importance of this observation was immense because the W and Z bosons were essential ingredients
in the SM.
One of the primary goals of DØ was to measure accurately many of the properties that characterize these fundamental particles. The high
energy and the intensity of the proton and antiproton beams at Fermilab make the Tevatron an ideal place to produce large samples of W and Z
events. During Run 1, DØ and CDF collected the world's largest sample of W bosons, with DØ accumulating over 100,000 W particles, a far cry
from the handful observed in their discovery. With such a large sample, DØ has made some of the best measurements of the properties of the W
boson, including its mass and couplings to other particles, as we briefly describe below.
W bosons are produced at the Tevatron mainly when a quark from a proton and an antiquark from an antiproton collide head-on at the DØ
detector. Almost immediately after being produced, the W decays into other particles within about 10-24 seconds. Roughly 10% of the time a W decays into an electron and a neutrino, and it is this decay mode that DØ uses to measure the W mass. While only one W boson with this decay signature is produced for about every forty million collisions, processes that mimic this decay are about 50 times less likely. Thus, although it took three years to accumulate the W events, the sample is nearly pure.
To extract the mass of the W boson, DØ first measures the momenta of its decay particles. The energy of the electron is measured in the
liquid-argon calorimeter. Since neutrinos rarely interact with matter, their momenta must be measured indirectly by invoking momentum
conservation. The sum of the momenta of all the particles produced in the collision (in the plane transverse to the proton and antiproton beam
directions) must be balanced by the transverse momentum of the neutrino. A quantity called the transverse mass of the W boson is then
calculated by combining the transverse momenta of the electron and neutrino, and the mass of the W is extracted from the shape of this
transverse mass distribution. The DØ value for the W mass is 80.482 ± 0.091 GeV, the world's most accurate measurement of this important
parameter published to date from any single experiment.
The experimental uncertainty of 0.091 GeV, or 0.11%, represents an improvement of about a factor of 100 compared to the original set of
measurements, and required an extremely detailed understanding of the experimental apparatus. For example, the mean calorimeter response to
the electron had to be known to better than one part in a thousand, and energy depositions as small as 100 MeV had to be taken into account in
collisions with up to 1.8 TeV (1TeV=106MeV) of total available energy. To put this in perspective, it is as if you had to know whether you had
several grains of sand under each of your fingernails when you weighed yourself on the bathroom scale. Luckily, Z bosons are produced in nearly
the same way as Ws, and their decay particles can be used to calibrate the detector. With 10,000 Zs available, DØ was able to understand the
apparatus to the required level of accuracy.
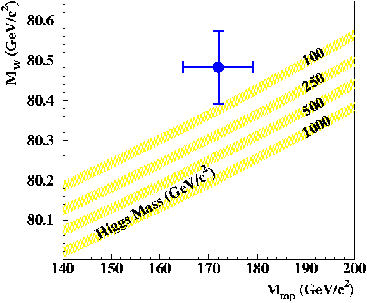
Fig. 7: Measured values of the top and W mass at DØ are shown superimposed upon predictions from the Standard Model in which the Higgs mass is varied between 100 and 1000 GeV.
The precision determination of the W mass, together with the mass of the top quark discussed above, can be combined to estimate the mass of
the Higgs particle. The W mass receives contributions from its virtual disassociations to top and antibottom quarks or to W boson and Higgs.
The properties of the Z boson, accurately measured at the CERN and SLAC e+e- colliders, also provide sensitivity to the mass of the Higgs. The full set of these measurements thus constrains the Higgs mass in the context of the Standard Model. Figure 7 shows the result of the DØ measurements. The indirect measurements using the Z, obtained mainly at LEP and SLC, and the directly measured top quark and W masses
from DØ and CDF agree well, and suggest that the Higgs boson has a mass below 200 GeV – perhaps within reach of the next run at the
Tevatron.
In addition to measuring the W mass, DØ used its large sample of Ws and Zs to probe the strength of the couplings between these gauge bosons
and the photon. The unified theory of electroweak interactions makes unique predictions for these couplings, which are quite different from
predictions one would derive from separate electromagnetic and weak theories. By studying events containing both a W boson and a photon, DØ
was able to show directly for the first time that the unified theory was indeed needed to describe the results. In addition, analyzing events
produced with a W boson and two jets allowed DØ to demonstrate directly that Ws and Zs interact with each other as predicted by the Standard
Model. Such tests of the couplings between the bosons probe the very heart of the electroweak theory, and any deviations from the predictions
would provide direct evidence of new physics. With some of the most sensitive measurements to date, DØ has been a world leader in studying
these couplings, but so far has found no sign of anything new beyond the SM.
While Run 1 was quite successful, the future for DØ is even brighter. When improvements to the Fermilab Tevatron and the DØ apparatus are
completed, DØ will begin to take data and expects to collect over 2.5 million W events. The uncertainty on the W mass will be reduced by at
least a factor of two, and significant improvements will be made in the measurements of the gauge boson couplings. Along with many other
interesting W and Z physics topics, the DØ experiment should be able to confront the electroweak sector of the Standard Model with
unprecedented sensitivity and with the hope and possibility of discovering something new.
< Back Next > - QCD PHYSICS
Return to For the Public main page
|