For the Public - Research Highlights |
Physics Highlights from the DØ Experiment (1992--1999)
Fermi National Accelerator Laboratory, Batavia, Illinois, U.S.A.
7. SEARCH FOR PHYSICS BEYOND THE STANDARD MODEL
It is an amazing feature of the Standard Model that, despite its extraordinary predictive power, it is almost surely incomplete.
There are 26 parameters needed to specify the SM, and these can only be supplied by experiment. The strong and
electroweak interactions that jointly make up the SM are seemingly unrelated entities; we would prefer to see a unification of
these forces but the SM does not do this. The mechanism that breaks the underlying symmetry of the electroweak
interaction, and thereby provides disparate masses to W/Z bosons and the photon, is not understood; in the SM the Higgs
boson is inserted to provide the symmetry breaking, but its mass is expected to be 1014 times larger than that of the W and Z
bosons unless some fantastic "fine tuning" is at work. Beyond these defects, the SM offers no clue as to why there are three
generations of quark and lepton families with nearly identical properties apart from their mass. It can accommodate, but not
explain the existence of CP violation, or why the cosmological constant that should be of order 10100 GeV is close to zero, or
how to get gravity into a unified framework with the other forces.
Twenty years of precision tests of this model have resulted in an enormous number of successful comparisons of data and
theory, with no verified departure from the SM. Despite this impressive predictive power, we firmly believe that the SM is
nothing more than a low-energy approximation to a more general theory, the one that explains our world in its completeness
and puzzling beauty. This is a very interesting situation, comparable to instances in the past that foreshadowed a major shift of
paradigm. Are we completely blind in our search for this more complete theory? The answer is "probably not". We have
several hypotheses that we consider as strong candidates for extensions beyond the SM. At the same time, it is imperative that
we look for any possible deviations from predictions of the SM, and the DØ experiment has been a pioneer in such studies.
One set of possible extensions of the SM, usually associated with a postulated new super-strong force involving new massive
families similar to the quarks, require the presence of particles called leptoquarks. The leptoquarks would have the properties
of both leptons and quarks, and thus would let quarks and leptons interact in a non-SM way. In 1997, the possibility of
existence of leptoquarks got a boost from experiments at HERA. By colliding positrons and protons, the HERA experiments
could produce single leptoquarks. In February 1997, the experiments H1 and ZEUS announced an excess of events over SM
expectations at large q2, with an invariant mass around 200 GeV, which could be interpreted as due to leptoquark production.
The evidence was not compelling, but the possible sighting could have had revolutionary implication, and it therefore set the
Tevatron experiments in motion to add information.
At DØ and CDF, leptoquarks can be produced in pairs via the strong interaction. This mechanism is well understood and is
relatively model independent. The high energy of the Tevatron offers the possibility of searching for leptoquarks to masses
higher than accessible at HERA. DØ physicists immediately teamed up for the search. It took three months of analysis to
unambiguously establish that the excess that HERA saw was not due to leptoquarks. DØ used advanced data-analysis
techniques, such as neural networks and other methods of multivariate analysis, introduced earlier in top-quark studies at DØ.
These novel techniques allowed DØ to establish the world's best limits on the existence of leptoquarks that could decay into
electrons and quarks. The lower limit on mass of the leptoquark from the DØ experiment alone was 225 GeV, more than
enough to rule out the possibility for the HERA event-excess of being interpreted as evidence for leptoquark production.
Combined with the 213 GeV limit obtained by CDF, the two Tevatron experiments were able to rule out the existence of these
particles with masses below 242 GeV. More general DØ limits on the mass of the first generation leptoquarks (MLQ), as a
function of the probability that these particles decay into electron and quark (b), are shown on the left side of Fig. 10.
Supersymmetry (SUSY) has been suggested as a possible cure for many of the shortcomings of the Standard Model.
Space-time symmetries such as those of translation or rotations of coordinates lead to momentum and energy conservation.
Supersymmetry postulates a further symmetry between bosons (integer-spin particles) and fermions (half-integer-spin
particles), thereby generalizing the Poincare group describing space and time. This radical reshaping of our understanding of
space-time is also a key ingredient in the theory of strings in multiple dimensions. When used as a phenomenological
ingredient of physics at the scale of present-day experiments, it provides a natural solution to the shortcomings of the SM
involving the instability of the mass of the Higgs boson, and permits the unification of the strong and electroweak forces.
Supersymmetry predicts that each known fermion and boson should have a mirror "superpartner" of the opposite type.
Clearly, supersymmetry is broken, since there is no spin-zero superpartner for the electron at 0.511 MeV. But to be
self-consistent, supersymmetry predicts that the superpartners should be found with masses below 1000 GeV, and some could
be within reach of discovery at the Tevatron.
The DØ experiment has searched for traces of supersymmetry in a variety of processes. So far, these searches have not been
successful, and have resulted only in limits on the existence of superpartners. Depending on the model parameters, squarks
and gluinos (the superpartners of quarks and gluons, respectively) with masses less than about 260 GeV have been excluded.
The right side of Fig. 10 shows the region of supersymmetry parameter space over which the DØ results have ruled out
squarks and gluinos. The parameters M0 and M1/2 refer to the unified masses of the spin zero and spin one-half
superpartners at the scale of unification of forces. Limits were also set on masses of charginos and neutralinos, the
superpartners of the W, Z, and Higgs bosons. Despite these negative results, hopes are high as capabilities for discovering
supersymmetry improve dramatically in the next Tevatron collider run. The mass reach will be about 100 GeV higher than
present limits on superpartner masses, bringing DØ into a very interesting range of SUSY parameter space.
Among other fundamental symmetries probed by the DØ experiment is the "broken" symmetry between the electric and
magnetic charges. We know that free carriers of electric charge exist, but there is no trace of a free magnetic charge, or
magnetic monopole. If monopoles exist, one would expect pairs of high-energy photons to be produced at the Tevatron at a
much higher rate than predicted by the Standard Model. This indirect search, though unsuccessful, yielded the most restrictive
limit on the mass of a possible magnetic monopole.
Recently, a novel idea was introduced for physics beyond the SM. It originates from string theory that views all known
particles as vibrations of tiny "strings" of energy. The recent success of string theory in explaining entropy flow in black holes
has drawn much attention. String theory, or its subsequent elaboration as membrane or M-theory, seeks to explain all physical
phenomena using structures in a universe with 10 or 11 spatial dimensions and time. The extra (beyond the usual four)
dimensions are believed to be "curled up" at a scale of at most 10-19 cm. However, recent suggestions predict that some of
these extra dimensions may be confined to a much larger scale, perhaps of the order of one millimeter. If this is correct, then
the highest energy scale we know of, the so-called Planck scale might be much lower than initially realized (~ 1 TeV, and not
1016 TeV). DØ is currently looking for possible manifestations of this predicted signal in several channels.
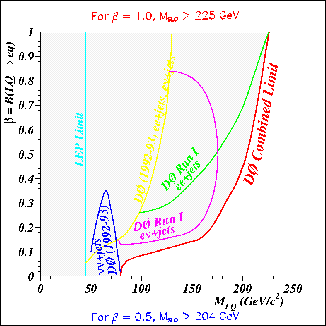
Fig. 10: Highlights of searches for new physics at DØ: limits on the mass of first-generation leptoquarks (left), and limits on squarks and gluinos in SUSY models (right).
The searches discussed above are just highlights of the many that DØ has performed in its very successful first run. DØ has
also looked for leptoquarks of other generations, additional quarks and vector bosons, quark-lepton compositeness, technicolor,
non-standard Higgs bosons, and more. We are closely following recent developments in theory, and several searches for the
manifestations of new theoretical concepts are still ongoing. Although no new physics has as yet been observed, DØ will
continue hunting for the unknown.
< Back Next > - CONCLUSIONS
Return to For the Public main page
|